Could a small fusion power reactor in Oxfordshire hold the answer to the energy crisis?
Fusion remains a long shot, but if they can pull it off none of the investors in Tokamak Energy in Culham will have to worry about money again, writes Steven Cutts
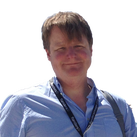
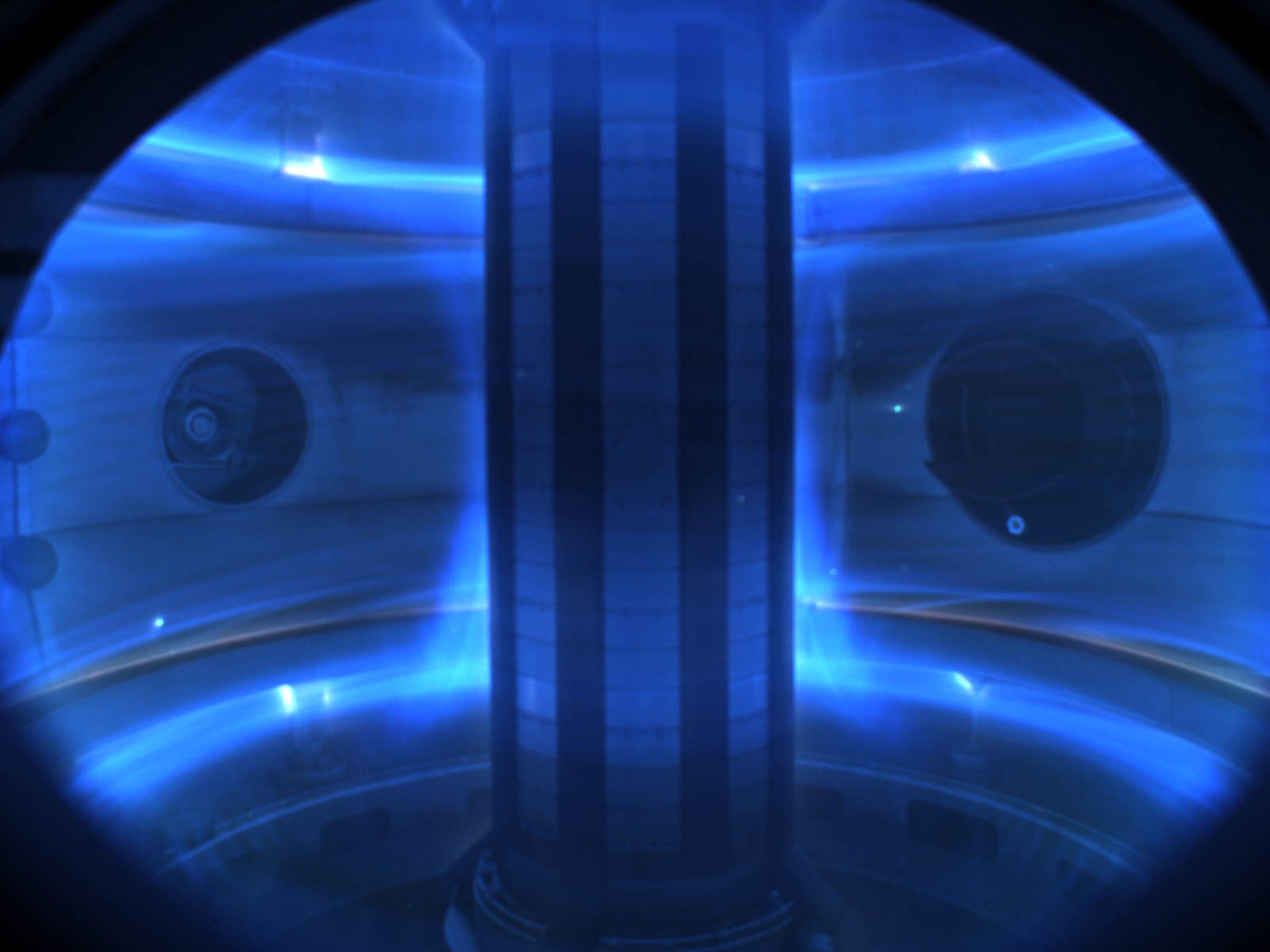
Energy remains one of the most powerful forces in the world today. It is what destroys our environment and constrains the growth of nations. In modern times, the politics of energy has driven us to war and provoked an entirely new political class, the environmentalists, who chastise us for the process of consumption and seek to exert some new kind of puritanical control. Energy is the new liquor that destroys our lives and we must all discard, less our addiction survives and our society doesn’t.
Unless, of course, we can solve all that with some new and fantastical scientific discovery that makes all previous forms of energy production redundant and might yet save mankind.
For most of the last 70 years this process has been fusion. Scientists still haven’t delivered, but that isn’t for lack of trying. So long and so hard has the quest for nuclear fusion become that for many, the prophecy has begun to wear thin. Fusion nuclear power is a mirage that recedes as fast as we approach it and the resources that have been so foolishly diverted into this impossibly expensive field might have been better deployed elsewhere.
The history of nuclear fusion is complex.
At the dawn of the 20th century, people still couldn’t decide whether atoms even existed. Following a few, rudimentary experiments, the entirely new field of nuclear physics soon emerged and went on to progress at breathtaking pace with all three subatomic particles being discovered at Cambridge University in England. Fusion was the magic that drove the stars but it wasn’t until after the Second World War that confidence in fusion as an energy source began to emerge. Again, the first attempts to build a fusion reactor were performed in our own country and with an optimism that would make Elon Musk turn red. Government officials announced that Britain’s man-made sun would soon replace all other forms of energy generation and change the world forever. It didn’t. Early fusion experiments actually consumed more energy than they produced.
After a series of failed projects, the British went on to persuade a number of governments to set up JET – the Joint European Torus – in Culham in Oxfordshire. That facility was completed in the early 1980s and is still in operation today. During the 1990s, JET actually set one of the existing records for a reactor of this kind but JET is approaching the end of its operational life. In the next few years it is due to be superseded by Iter, a next generation fusion facility slowly taking shape in the south of France.
Needless to say, none of this is cheap. By 2050, Iter will have set us back by at least £50bn, although, to be fair, the cost will be shared by many governments around the world. Like JET, Iter will be unable to produce a single watt of electricity. These reactors are merely stepping stones to the first commercially viable fusion reactor that may or may not become credible at a future date.
But for all the delays and setbacks, the quest for fusion is not finished. There are a number of much smaller, upstart projects around the world all hoping to do the same, sometimes on a far more limited budget. One such project is now underway near Oxford where Tokamak Energy has already constructed their own device. Tokamak is functioning on a budget of barely £200m and when viewed besides Iter, it barely registers on the Richter scale for big science. What are the chances of the smaller rivals actually breaking through and finally delivering the idealised energy source that man has craved for so long?
At 5 by 4 by 3 metres, the Tokamak reactor it is quite limited in size and designed around a different principle to both JET and Iter. Whereas traditional fusion research has depended on a torus or doughnut shaped reactor, Tokamak has built a spherical device that has been funded – at least in part – by the private sector. An idea that seemed so outlandish and expensive that only the taxpayer could be expected to pay for it is suddenly attracting start-up capital of its own. Why? Because we’re almost there? Or because of the sheer scale of the riches that might yet stem from this field? I suspect probably a combination of the two. Fusion remains a long shot, but if they can pull it off, none of the investors in Tokamak will have to worry about money again.
Another unexpected development of fusion is the arrival of Covid-19. Having arranged to visit the Tokamak facility in person, I found myself excluded by the virus but with the help of some video conferencing software, I was able to speak to one of the co-founders of the company, Doctor David Kingham.
In the first instance, I had a philosophical question. There are a lot of new energy sources out there, but what advantages would a fusion nuclear future provide?
Given the scale of the technical challenges involved, the early reactors are unlikely to be cheap. It is not even the case that a future fusion reactor would eliminate the issue of radioactivity. However, most experts agree that the volume of radioactive material produced would be much smaller than current day fission reactors. What material a fusion reactor would produce will decay and effectively become safe in about 100 years. That might sound like a long time, but try to bear in mind that the nuclear waste being produced by our current reactors is expected to remain toxic for many thousands of years and the issue of waste disposal remains a major headache for the proponents of nuclear power everywhere. Nuclear fusion has the additional advantage that it wouldn’t produce the kind of by-products that might enable the occasional rogue states in this world to build their first atomic bomb. Fusion also scores well on that other popular disaster movie concept: meltdown. That could happen with nuclear fission but it just couldn’t happen with fusion.
Renewables are improving but they represent an intermittent and often unpredictable source of power that appears to supplement rather than replace existing technology
As Doctor Kingham explains, funding for nuclear fusion research always comes with a sense of urgency although the nature of that urgency tends to change over time. In the 1970s, it was seen as a potential response to the Arab oil shock. Later, in the aftermath of the Three Mile Island and Chernobyl disasters, it seemed to offer all the advantages of nuclear power without the issue of radio activity. Today, it finds itself presented as a solution to global warming.
Conventional nuclear power has been around for decades and by rights its cost ought to be coming down. It isn’t. Nuclear power is increasingly burdened by costs that appear to be increasing. Ongoing concerns about safety and an emphasis on regulation have made it more difficult to build nuclear although the political acceptability of fission nuclear power varies wildly between countries.
Kingham lists a few of the funding sources for this project, mentioning that his own company – Tokamak Energy – has just received a government grant of £500,000. Funding from the taxpayer is a long-standing feature of nuclear research, but the fact that they’re picking up private sector investors as well is more than astonishing. They already employ about 120 people and actually have a prototype up and running.
The Oxford group aren’t the only challenge to Iter. There are other, relatively small-scale projects underway, one of them supported by MIT in the US. Each of these groups is trying to circumvent the issue of finite resources with the use of innovative new technologies, in particular the generation of powerful electromagnetic fields by hot superconductors. The US-based Commonwealth Fusion Systems is planning a compact fusion reactor using a high temperature superconductor made of ceramic-like material. With luck, it may be able to generate 20 teslas.
The Americans are spending this kind of money in parallel with their commitment to the Iter project and they wouldn’t be doing it if they didn’t think there was at least a chance that they might succeed. At a recent speech in France, President Emmanuel Macron and the leaders of the Iter project were questioned about the rival projects and openly acknowledged that such projects might usurp their own. Iter has been so long in the offing and so committed in its scale that they had to go ahead with the traditional torus concept anyway.
Why then do the people at Tokamak think they can still finish first in a race against JET and the forthcoming might of Iter?
JET has previously run at a peak energy of 16MW. The scientists involved adjusted the operation for a peak of one second. “If running commercially,” says Kingham, “they’d have to maintain this every day.” This is a giant leap of the imagination but the key here might lie with magnets. “The copper wire surrounding JET created 3 tesla at the centre of the plasma and a maximum of 9 telsa at the magnet itself.”
Nuclear fusion is the driving force behind the stars but it isn’t easy to replicate the environment of a star in a machine
All fusion reactors attempt to mimic the physics of a star by heating the gas inside them to a fabulously high temperature. At these temperatures, the electrons are torn off their parent atoms leaving the naked atomic nuclei free to swim in a sea of disconnected electrons. Such a gas is described as a plasma and since all the particles within a plasma carry an electrical charge, it can be controlled and manipulated by an externally applied magnetic field.
Tokamak uses high temperature superconductors that surround a spherical reactor. Many years ago, the Soviets came up with an idea for a fusion reactor that was shaped like a doughnut and invented a suitably polysyllabic Russian name. Virtually all fusion reactor designs since then have been built around the doughnut concept. JET – perhaps the most successful fusion reactor to date – was designed in the 1970s and continues to perform cutting edge research.
In more recent years, liquid helium-cooled superconductors have made an appearance in quite a few pieces of engineering, massively increasing the strength of magnetic field on offer. A liquid helium-cooled superconductor will retain its magnetic field until the helium runs out and their temperature rises above a critical level. At that point, their magical properties cut out and the magnetic field disappears very abruptly. In short, superconductors are exotic and they cost a fortune.
However, during the early 1980s, scientists discovered a group of ceramic compounds that could super-conduct at much higher (although still very cold) temperatures. Iter will use liquid helium-cooled superconducting coils operating at about four degrees above absolute zero. The strength of their magnetic field here be around 12 tesla, massively superior to copper wire. In contrast, both MIT and Tokamak are using so-called “hot” conductors capable of operating at about 20 degrees above absolute zero and generating as much as 24 tesla.
Given that hot superconductivity was discovered in the early 1980s, why is it only just beginning to replace liquid helium-cooled magnets? Doctor Kingham’s explanation is simple: “Only in the last 10 years has the manufacturing capability scaled up to make hot superconductors an option.”
Nuclear fusion is the driving force behind the stars but it isn’t easy to replicate the environment of a star in a machine. The raw subatomic particles within the reactor collide at incredibly high energy and speed and occasionally fuse together to produce a larger particle. Two hydrogen atoms bind together to produce a helium atom. This process releases an immense amount of energy and at least part of that energy manifests itself in a spare neutron that punches its way out of the reaction with fantastic energy. In fact, two thirds of the energy produced is given to the neutron. Neutrons don’t have an electrical charge and cannot be manipulated by magnetic fields. They fly straight through the wall of the reactor and are viable for absorption by the design engineers of a fusion reactor.
The pressure present at the centre of our sun is so vast that it defies our imagination. In contrast, many modern fusion reactors here on Earth are operating a plasma at a pressure of about one atmosphere. To replicate a process that occurs spontaneously in the heart of a star, we need much higher temperatures than those found in the centre of the sun. The temperatures in a fusion nuclear reactor are a staggering 100 million degrees centigrade. In this environment, electrically charged particles are trying to collide at a speed that is difficult to comprehend.
What are the chances of the smaller rivals actually breaking through and finally delivering the idealised energy source that man has craved for so long?
When we start to think about fusion in these terms we begin to understand why our attempts to harness the plasma have taken so long.
Hydrogen is the first atom in the periodic table. It contains just one proton. When two hydrogens fuse together, they form the second atom in the periodic table: helium. This reaction also produces a spare neutron. The actual weight of these fragments is very slightly less than the weight of the two hydrogens we started out with and the missing weight has been converted into pure energy. The return on the investment being defined by Einstein’s iconic formula, E=mC2.
“Eighty per cent of the energy is in the neutron, and 20 per cent is in the helium. At these temperatures the helium atom is ionised. It carries an electrical charge and we can control it using an externally applied magnetic field.” In contrast, the high energy neutron flies straight through the reactor wall and – hopefully – impacts on a dense lithium blanket that the scientists intend to wrap around the reactor. With millions of neutrons colliding with the blanket every second, the lithium becomes hot and we can use this to heat water and drive steam turbines.
One fortuitous side effect of this process is the production of tritium, an isotope of hydrogen that can be separated from the blanket and used as a fuel for future reactions.
If any of this sounds too good to be true, it is. The technology required to build a lithium blanket still isn’t out there. As Doctor Kingham explains, “it would be a continuous flow process to extract a liquid blanked concept or a bubble bed blanket. We don’t have a programme demonstrating that yet so the current device, there isn’t a liquid blanket.”
It will be 2025 before anybody even needs a lithium blanket and the fastest they could possible produce a commercially credible reactor is 2030. Building a lithium blanket isn’t the hardest thing about fusion nuclear power and they can always develop one later.
Talking to Doctor Kingham and looking at the new work around the world it’s obvious that there’s a renewed interest in fusion nuclear power. In fact, many in the plasma physics community never really lost faith in the concept and like most technologies, knowledge has been gained by incremental steps and grown quietly and behind the scenes. But there’s got to be more to it than that. I ask him why he thinks we can succeed now where his predecessors have so clearly failed.
“It isn’t one thing.” Maybe the high temperature superconductors and previously unimagined magnetic fields that stem from this work. Modern day tech companies in Silicon Valley have demonstrated that the private sector can create a momentum that governments struggle to match. A salaried civil servant with a gold-plated pension isn’t the most dynamic person on this planet. Government projects are hindered by bureaucracy. They struggle to gain purchase.
The new Tokamak reactor is four metres tall and three metres in diameter. As things stand, it doesn’t quite achieve energy gain but they’re pushing to correct this problem.
Whilst the fusion community battles on from one decade to the next, the rest of the scientific people in the world have been looking elsewhere. Over the past few years the price of a photovoltaic panel has plummeted. It has been suggested that by 2050, as much as 30 per cent of global electricity could be obtained from the sun. Large scale solar power looks set to provide energy independence to countries that previously had none. Given that solar power already exists and given that costs are falling so rapidly, why do we even need fusion?
“Renewables are improving but that they represent an intermittent and often unpredictable source of power that appears to supplement rather than replace existing technology. Carbon capture technology – another hot topic in energy research – seems likely to disappoint if we attempt it on a higher scale.” In practise, a “30 per cent gap in world energy needs is still up for grabs by 2050”.
But the fact that there is a crying need for nuclear fusion doesn’t in itself guarantee that it will become a reality, even in the presence of new and more elaborate funding. We can’t plan for a future on the basis of a technology that does not yet exist.
And yet the field continues to advance and there are reasons for optimism. “Fundamental advances in materials science, plasma physics and better magnets all point to a brighter future. In particular, we have seen an exponential increase in computer technology. Computational technique has also improved with the introduction of very large computers, parallel processing or web-based computing capabilities.
Some American groups are talking about a pilot fusion plant within 10 years. I’ll believe it when I see it, but I hope they’re right. In British culture, the Americans are the endless butt of jokes but they have a habit of developing new technologies that actually work.
If it does, the next generation may spend a lot of time tearing down windmills and ripping off solar panels. The advantages of fusion-derived power are difficult to exaggerate and could do a lot more than quench our need for electricity. A society that could electrolyse sea water whenever it wants could easily introduce a hydrogen economy. Not having to fight over energy resources won’t solve everything but it will do a lot to secure our collective future.
Subscribe to Independent Premium to bookmark this article
Want to bookmark your favourite articles and stories to read or reference later? Start your Independent Premium subscription today.
Join our commenting forum
Join thought-provoking conversations, follow other Independent readers and see their replies
Comments